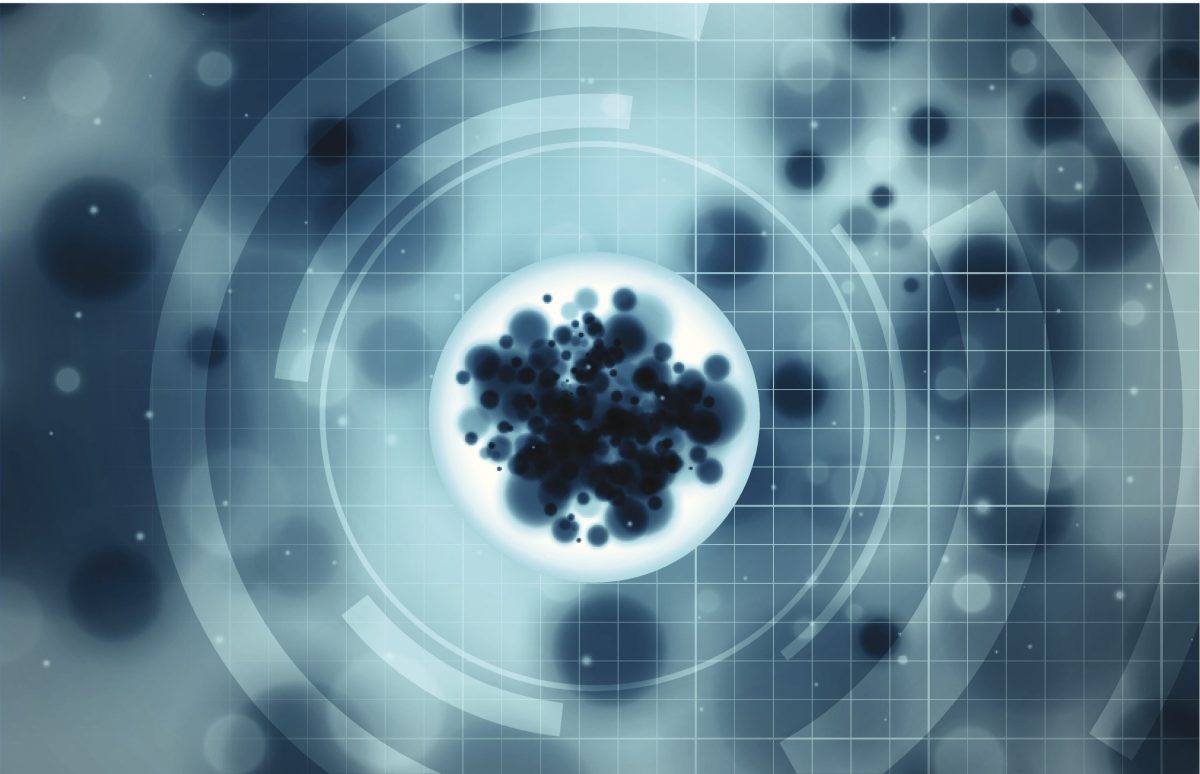
Abstract: Advances in technology, particularly biotechnology, over the past decade have dramatically changed the world. Scientists are employing gene editing tools to cure genetic diseases, reduce the effects of climate change, and generate sustainable food sources. These same tools, however, can be used to modify pathogens to develop and deploy novel biological weapons. The nature of these tools and our understanding of specific viral genomes makes this process tunable. Components of a disease such as transmissibility, lethality, and the infectious window can potentially be modified for desired tactical, operational, or strategic effects. While this capability is currently beyond the skills and knowledge of the biology enthusiast, a trained individual would possess such skills and knowledge, though they may lack the necessary material and infrastructure support. Hence, it is necessary to develop and maintain capabilities that can respond to a variety of pathogens and possible effects.
The emergence of the SARS-CoV-2 virus in Wuhan, China, in November 2019 and its subsequent worldwide spread has had tremendously destabilizing effects, which are still being felt more than two years later. Lessons from COVID variants include immediate impacts at the local level (initial variant), global pandemic effects from the Delta variant to include significant and protracted economic impact, and the more sub-lethal, sustained economic, political, and healthcare impacts of the Omicron strain. The global SARS-CoV-2 pandemic has also highlighted the ongoing biological revolution that has resulted in the rapid development and employment of new diagnostic tests, vaccines, and other targeted treatments including monoclonal antibodies and antiviral drugs. Over the past decade, the intersection of technology (e.g., computer science, automation, DNA sequencing) and biology has expanded exponentially, becoming embedded in economies and society. This intersection, along with the demonstrated impacts of SARS-CoV-2, is fraught with opportunities and risks. The tools for curing genetic diseases, reducing the effects of climate change, and generating sustainable food sources are now being developed and tested. Yet, these same gene editing tools could be employed to generate and modify biological weapons, making it important for both the counterterrorism community and scientific community to anticipate how the scientific advances may change the bioterrorism threat landscape.1
In this article, the authors consider the theoretical potential for bioterrorists to select a viral platform and genetically modify viral transmissibility, incubation and infectious time windows, and lethality along with the manner of death, creating what are in essence tunable bioweapons. Such bioweapons could achieve targeted effects tailored to timescale, physical and psychological effect, with intended tactical, operational, and strategic levels of impact, with the most impactful viral agents producing all three effects.
To anticipate the potential future threat posed by tunable viral agents, the article first examines the advancing biotechnological toolkit that bad actors may be able to exploit. It then delves into the singular threat posed by viral agents compared to other potential forms of weaponized pathogens such as bacteria, with the COVID-19 pandemic underscoring the threat posed by highly transmissible viruses. The next section describes how biotechnology tools allow for the bioterrorist to select a viral “chassis” and then prospectively genetically tune the respective system variables of lethality, transmissibility, and infectious window for tactical, operational, or strategic effects, or, to maximize impact, combinations thereof. The piece then discusses the duality of emerging biotechnology tools for developing and deploying potential bioweapons as well as their countermeasures. The article closes with some concluding observations.
The Advancing Biotechnology Toolkit
While there is a variety of biological gene editing tools, perhaps the most notable is the Clustered Regularly Interspaced Short Palindromic Repeats (CRISPR) Cas9 system.2 This system comes from the adaptive immune response of bacteria to prevent viral infection. During the process of viral invasion, the virus hijacks the host cell to replicate its genetic material to make multiple copies of itself to infect other cells. Bacteria utilize the CRISPR system to identify the invading viral genome and to subsequently cut the viral genetic material using the associated Cas9 protein. The critical capability of the bacterial CRISPR-Cas9 system is the recognition of the pathogen genome using a target sequence that is complementary to a portion of the target viral genome. The CRISPR can then quickly identify a known invader’s genetic code and rapidly digest and incapacitate it upon recognition.
The great technological leap of CRISPR is its application to edit plant and animal genomes. With several advancements in biotechnology, including rapid and affordable whole genome sequencing and nucleic acid (DNA and RNA) synthesis, both scientific investigators and prospective bioterrorists with the requisite scientific knowledge and equipment can now with relative ease design and manipulate specific target sequences to modify, insert, or delete portions of the genetic material. The ease of ‘copy/cut/paste’ modifications of specific genetic sequences has the potential to cause either a loss or gain of biological function. While the potential impact on human diseases can be tremendous, as evidenced by recent news of a patient being possibly cured of HIV infection,3 so too can the negative consequences. In 2018, Dr. He Jiankui, a Chinese biophysicist, employed CRISPR to delete a portion of the CCR5 gene in embryos with at least one HIV-positive parent. The CCR5 gene “encodes a protein that allows HIV to enter immune cells,” and a small deletion (CCR5-delta32) therein had been previously shown to protect cells from HIV infection.4 This use of CRISPR not only shocked the world but was also undertaken without the consent of the Chinese government. Jiankui was subsequently found guilty of “illegal medical practice” and sentenced to three years in prison, while several of his colleagues received shorter sentences.5 Even though Jiankui was an established scientist, his employment of CRISPR to edit human embryos less than six years after the 2012 Science article by Jennifer Doudna and Emmanuelle Charpentier first reported the programmable nature of CRISPR illustrates its relative ease of use.6
The combined lessons of the COVID pandemic, along with an increasing effective biotechnology toolkit, add to a possible playbook for bioterrorists who seek to use viral platforms to achieve effects along a continuum of targeted, local endemic effects all the way through to inducing a global pandemic. This playbook might also be leveraged by state actors or state actors through proxies.
The Singular Threat of Viral Agents
The suite of viral outbreaks in the 21st century, including COVID-19, Ebola, Zika, SARS, MERS, swine flu, and avian flu, readily highlights the dangers of these highly transmissible agents. When factoring in their respective varying lethality, routes of infection, and overall infectivity, viral agents clearly pose a considerable security threat. However, the most well-developed biological warfare agents have historically been bacterial. Bacillus anthracis, the etiological agent of anthrax, has been studied extensively and developed both at home and abroad as a potential offensive bioweapon, largely due to the microorganism’s ability to exist almost indefinitely as inert spores. There have been multiple instances of the intentional release of anthrax spores and millions of research dollars spent on developing antibiotics to potentially treat inhalational anthrax.7 Similarly, Francisella tularensis, the causative agent of tularemia, sometimes known as “rabbit fever,” has been similarly studied extensively for both offensive and defensive purposes.8
But bacteria and viruses are completely different. First and foremost, bacteria are a domain of life. Bacteria are microscopic living organisms, normally existing as single cells that contain the essential biomolecules—sugars, proteins, lipids, and nucleic acids—and are fully capable of reproducing according to their respective genetic codes. Viruses, on the other hand, are not living organisms. Rather, they are variably comprised of a nucleic acid, DNA or RNA, that encodes for a small number of capsid coat proteins and virus-specific enzymes. They are obligate intracellular parasites that are only capable of replicating within other organisms. Viruses are known to infect all types of living organisms, from bacteria through plants to animals, hijacking the machinery of life to read their genetic code and produce proteins that aid in their propagation within an organism and inevitably to the next organism, via infection by many different mechanisms.
Therefore, it is imperative to remember that bacterial threats are not viral threats. They are completely different. Bacteria are several orders of magnitude larger than viruses, incredibly more complex, diverse, and must be provided nutrients to stay alive. Viruses, on the other hand, along with their appropriate host cell must be maintained to survive and propagate. Bacteria that produce spores as evolutionary adaptations to survive in nutrient-poor conditions lend themselves to large-scale production and an ability to infect on a relatively large scale. Otherwise, in a laboratory setting, bacteria are typically stored either in solid or liquid media, wherein they pose little to no harm. Viruses, due to their extraordinarily small size and lack of complexity, are much easier to spread via surface contact or in the air, either intentionally or unintentionally, and depending on the diseases they cause may be appropriately categorized as much more dangerous than bacteria. Common and uncommon bacterial infections are nowhere near as transmissible as most viruses due to their larger size and relative inability to be effectively aerosolized and passed from organism to organism via coughs and sneezes. This is illustrated by the etiology of pneumonia infections where one study showed 46.4 percent were viral, 14.4 percent were bacterial, and 25.4 percent were co-infections of both virus and bacteria.9 By most measures, bacterial infections are localized and commonly transmitted via direct contact, water, insect vectors, or small animals. Viruses, on the other hand, know little of such boundaries.
Finally, drugs developed to treat bacterial infections are known as antibiotics and either stop bacteria from reproducing or outright kill them. Drugs developed to treat viral infections are known as antivirals and typically either disrupt host cell mechanisms or target the hallmark nucleic acids and proteins of the disease-causing viruses. There is also a variety of broad-spectrum antibiotics that can kill a wide range of bacteria, while antivirals typically are targeted to a small number of viral species, at most. Both due to their completely different targets, as well as the fundamental difference that bacteria are living organisms and viruses are non-living replicative units, antibiotics and antivirals are fundamentally not interchangeable. The differences in infectivity and treatment make viruses a logical choice as a starting “chassis” to design a bioterror weapon.
Tailoring the Attributes of Viral Agents for Intended Effects
The process for biological design is simple and relies on the genetic sequence of the target pathogen. Within the genetic code of viruses and eukaryotic cells are two distinct regions known as exons and introns. During the transcription process, where the genetic material (DNA or RNA) is converted to messenger RNA, the introns are spliced out and the exons encode for specific proteins. To edit the genetic code, it is critical to understand these regions since editing a non-coding region will have little to no effect on the function of the process. Depending on the pathogen, information about exons and introns may not be known or at the level of detail required. Editing the genetic code also requires understanding the desired effects of the manipulation in terms of gain or loss of function. These desired effects may require the simple deletion of a portion of the genetic code or the slightly more complicated insertion of a genetic sequence. The CRISPR-Cas9 system enables both options and requires the correct configuration guide RNA prior to the actual development of the pathogen.
Biotechnology tools allow for the bioterrorist to select a viral “chassis” and then prospectively genetically tune the respective system variables of lethality, transmissibility, and infectious window (see Figure 1).10 The availability of these tools reduces many technical hurdles. However, the development of a biological weapon through substantive modification of any sort of virus requires the bioterrorist to have considerable knowledge and awareness about both the virus and the desired outcomes. The relationship between viral genome and desired system variables to achieve bioterror effects may not be clearly understood today, but the rapid development of biotechnology tools and scientific understanding portends the elucidation of these structure-function relationships in an ever-expanding toolkit that could just as easily serve the common good as it could serve purposely nefarious intentions.
Figure 1: The 3D plot illustrates relationship between desired effects (tactical, operational, and strategic) and tunable factors (X-axis: lethality/death rate, Y-axis: transmissibility, and Z-axis: infectious window). Lethality is the ratio of deaths among infected individuals. Transmissibility is the infectivity of the pathogen or how easy it spreads. The infectious window is the period of time between the point of infection and when the pathogen is reliably detectable, with a short window (a low Z-axis value) meaning fast detection and a long window (a high Z-axis value) entailing slow detection and more severe public health impacts. As previously mentioned, operations are a continuum of three categories: tactical, operational, and strategic. A tactical to strategic effect (right, upper, back box) means a viral agent can be expected to produce an effect end point that is tactical, operational, or strategic with lower-tier outcomes inclusive. By examining the relationship and interconnectivity of the tunable factors, it is possible to determine the desired effects. Conversely, it is possible to identify the levels of the tunable factors to achieve the desired effect level.
Tactical to Strategic Bio-Pathogens
U.S. military operations are a continuum of three categories: tactical, operational, and strategic.11 The tactical level of war can be viewed as battles and engagements at the unit or task organization level such as division or corps.12 The operational level builds upon the tactical level and is the level of war where campaigns and major operations are planned to achieve strategic objectives, generally occurring at the corps to field army level.13 These campaigns and operations are generally conducted at the theater level. The strategic level is greater and occurs when a nation employs its national resources to achieve the nation’s security objectives. From a military perspective, this can entail a corps to joint force land component commander.14 At the strategic level, a nation can be part of a multinational coalition.15
These definitions of military operations can also be used to describe and understand the effects of a bioterrorist threat. A biological weapon that only produces tactical effects would be one limited to a local or confined area. For example, in 1984, the Rajneeshee cult “contaminated salad bars at 10 restaurants” with Salmonella to sicken the local population of The Dalles, Oregon, in order to influence the county elections. This relatively simple attack led to 751 reported cases of Salmonella poisoning in a county where there are typically fewer than five per year.16 The 2001 Amerithrax attack could be considered a biological attack with primarily operational and strategic effects. The fact that multiple letters were sent to political leaders and journalists in multiple states created operational effects. Letters sent over a two-month period achieved strategic effects by disrupting the federal government as it endeavored to recover from the September 11th attacks and amid emerging operations in Afghanistan. While it could be argued that the letters did generate tactical outcomes, in terms of isolating buildings and personnel, the detection of Anthrax immediately elevated the issue beyond local control to the state and federal governments.
Along these same lines, the SARS-CoV-2 pandemic is a biological pathogen incident that has been full spectrum, from tactical to strategic effects, with the disruption of whole economies and concomitant political and social unrest (e.g., mask mandates and lockdowns). Specifically, it was reported in July 2021 that the U.S. economy had contracted by nearly 20 percent from the fourth quarter of 2019 to the second quarter of 2020.17 At a tactical and operational level, the healthcare system was overwhelmed in many areas, forcing assets to be shifted between states and requiring the support of FEMA and the National Guard to set-up field hospitals.
Depending on the objective a bioterrorist may have, a virus can be modified to achieve tactical, operational, and strategic end states or combinations thereof. Selecting a virus is perhaps the first critical step. Merely selecting a virus is an academic process and does not mean that the bad actor has access to or the ability to acquire purified quantities of the virus to be modified. Some of the elements to be considered are inherent transmissibility, infectious window, and lethality. The infectious window is the period of time between the point of infection and reliable detection of the pathogen and is often confused with the incubation period, which is the time elapsed between infection and the onset of symptoms.18 These components may be directly tied to the overall desired tactical, operational, or strategic effects, and selecting one that most closely aligns with the overall objective reduces the amount of modification required. For example, modifying a seasonal influenza virus to achieve the same hemorrhagic fever hallmarks of an Ebola virus would require significant modification to the viral genome that may not produce a viable virus.
After a virus is selected, it would be necessary for the bioterrorist to modify the genome to achieve the desired effects. This design process is not easy and requires an in-depth knowledge of the viral genome and which sequences encode specific proteins. The biochemical process of inserting a gene is easy, but designing the sequence, knowing where to insert the sequence, and ensuring that the sequence does not affect other parts of the genome that encode for proteins is critical.
To imagine this selection and design process, the cube in Figure 1 highlights the relationship between the attributes of the modified pathogens (inherent transmissibility, infectious window, and lethality) and tactical, operational, and strategic effects or, for the most impactful viral agents, a combination of those effects. For example, a virus with low transmissibility, short window before detection, and high lethality rate might be employed as a tactical weapon, and shifting the factors of transmissibility and infectious window would shift a tactical pathogen to a weapon that produces operational or strategic effects or both. Manner of death would also amplify psychological effects, potentially elevating what would otherwise have been merely tactical outcomes to also include operational or even strategic effects, allowing for broader and longer-lasting effects from local execution of bioterrorist acts. For instance, a dramatic, gruesome public manner of death involving significant blood profusion from bodily orifices would heighten the perception of a threat, compared to victims quietly expiring from low blood oxygenation out of sight in a medical facility.
As previously mentioned, development and implementation of modified pathogen requires sophisticated knowledge and capabilities. An April 2019 CTC Sentinel article highlights the relative difficulty for a non-state actor to develop a modified pathogen bioweapon, as illustrated in Figure 2.19 While a nuclear weapon is the most complex and difficult weapon of mass destruction to develop due to the constraints of acquiring fissile material, biological weapons do not have similar acquisition limitations. Quite literally, bacteria and viruses are everywhere. Transforming relatively benign bacteria and viruses in significant quantities is difficult, as it requires infrastructure, knowledge, and technical skill.20 Infrastructure relates to controlling the environment for optimal growth and modification. This also includes personal protective equipment, such as gloves, masks, and suits, along with supplied air and hoods as necessary.
While the necessary infrastructure and equipment can be acquired through legitimate and non-legitimate channels, knowledge and technical skill are more difficult. Reading journal articles can provide information about a virus or a method, but it is not the same as knowledge or technical skills that are only achieved through laboratory experience. It is the gap in knowledge and technical skill that drastically increase the complexity of viral and bacterial biological agents (e.g., Smallpox and anthrax, respectively) compared to biological toxins (e.g., ricin),a as shown in Figure 2. Compared to the general population, those individuals with said skills and knowledge are relatively few. However, advances in biotechnology, coupled with the democratization of gene editing tools, are slowly but steadily diminishing this barrier.
Figure 2: The relative complexity for weaponization and likelihood of use for nuclear, biological, and chemical weapons. The red dotted line and red arrows highlight that space in which non-state actors currently operate. HME refers to homemade explosives and TIC refers to toxic industrial chemicals, such as chlorine.22 As previously highlighted, biological toxins are chemicals/compounds that are extracted from biological organisms such as ricin and botulinum. The pathogen biological agents are bacterial and viral requiring greater knowledge, infrastructure, and other materials to develop and deploy. The gaps should be visualized as a continuum. For example, non-state actors have to overcome the elements of gap 1 as well as gap 2 in order to be able to use a nuclear weapon.
Medical Countermeasures
Just as the bioterrorist’s ability to potentially tune and tailor viral agents for enhanced lethality and other means of disrupting society continues to increase, so too does the ability to rapidly develop and utilize a scalable defense. Medical countermeasures broadly include three lines of defense: detection via diagnostics, treatment via therapeutics, and prevention through vaccines. More specifically, they are categorized as “medicines and medical supplies that can be used to diagnose, prevent, or treat diseases related to chemical, biological, radiological, or nuclear (CBRN) threats.”21
The ongoing COVID-19 response has highlighted the U.S. government’s capabilities for responding to a novel viral agent. Precision, accuracy, and availability of detection kits continues to be a critical frontline method for identifying infected individuals. Classical medicine itself provides a standard middle barrier. Then, experimental therapeutics, such as convalescent plasma, antibody cocktail treatments, and more traditional small molecule antiviral drugs, have all made their way into popular conversations as a final line of defense against advanced disease progression. But ultimately, the greatest defense against a novel viral agent continues to be vaccination, and the COVID-19 response has showcased extraordinarily successful private-public partnerships that have yielded multiple FDA-approved vaccines, which have been made widely available to nearly the entire U.S. population in a matter of months.
One can then reasonably conclude that subsequent viral events, possibly of a bioterror nature, will demand an even faster and more impressive response from the whole of U.S. government in terms of diagnostic testing, therapies, and care modalities. This precedent to rapidly develop therapies perhaps began during the response to the Ebola outbreak in 2014-201623 and has continued more recently as drugs to treat Ebola have obtained both normal and special FDA approvals through military partnerships.24 The development of medical countermeasures that are tailored to treat novel viral bioagents, however, will inevitably lag behind the initial deployment of such novel agents. Sustained anticipatory development of broadly applicable countermeasures, especially at the strategic level, is required to react to novel agents.
Conclusions
The sophisticated bioterrorist, using common biotechnology tools, may be able to tune transmissibility, infectious window, and lethality rates to achieve tailorable effects at the tactical through global strategic level. However, such individuals and organizations are currently rare and, based on their scarcity, can be targeted through their support networks, which provide material, information, and infrastructure.25
Anticipating threats and the methods used to develop such threats continue to place a premium on the importance of comprehensive and rapid detection and mitigation strategies. Such detection strategies might include massively networked wearable biometric sensors embedded in smart watches and sensor devices such as those under development with the Defense Threat Reduction Agency (DTRA) and Defense Advanced Research Projects agency (DARPA).26 Rapidly developable mRNA vaccines and monoclonal antibody therapies offer a starting point for relatively rapid mitigation, but are currently still far too slow to prevent a bioterrorist’s desired effects.
The SARS-CoV-2 pandemic has highlighted the global impact of one viral pathogen, but the tools to modify and tailor countless other viruses for a specific target and effect currently exist. Efforts must be undertaken to stay ahead of how these threats are applied. This includes using the same technology and knowledge to design rapid medical countermeasures and detection equipment. This effort begins with a comprehensive strategy for not only the U.S. government and the Department of Defense, but also allies of the United States as viruses do not recognize international borders. As preventing the development of biological weapons by non-state actors is increasingly difficult, domestic and international policies, funding, and organizational resourcing must coalesce to match the speed of science. CTC
Major Stephen Hummel is currently a Ph.D. student in the Biology Department at Boston College. Previously, he served in both Iraq and Afghanistan and as a USAREUR CBRN Plans Officer, an Assistant Professor in the Department of Chemistry and Life Science at USMA, a Nuclear Operations Officer on a Nuclear Disablement Team, and most recently as the Deputy, Commander’s Initiatives Group at 20th CBRNE Command.
Colonel F. John Burpo currently serves as the Head of the Department of Chemistry and Life Science at USMA. As an artillery officer, he served in airborne, armor, and Stryker units with humanitarian, peacekeeping, and combat operational deployments. He also served as the Deputy Commander-Transformation for the 20th CBRNE Command. He has a Sc.D. in Bioengineering from the Massachusetts Institute of Technology.
Lieutenant Colonel Jeremy R. Hershfield is a Medical Service Corps officer and currently the Chief of the Core Laboratory at Brooke Army Medical Center. He previously studied biothreat bacteria at USAMRIID, managed a research portfolio of biothreat virus and bacteria at DTRA, and was an Assistant Professor in the Department of Chemistry and Life Science at USMA. He has a Ph.D. in Molecular and Cell Biology from the Uniformed Services University of the Health Sciences.
Lieutenant Colonel Andrew Kick is an Academy Professor in the Department of Chemistry and Life Science at USMA with expertise in viral pathogens and immunology. He earned his Ph.D. in Comparative Biomedical Sciences from North Carolina State University. As an Army officer, he held CBRN, Military Intelligence, and Nuclear/CWMD positions at the company through the division-level.
Dr. Kevin J. O’Donovan is an Associate Professor in the Department of Chemistry and Life Science at USMA with expertise in neural development and axon regeneration. He earned his Ph.D. in Neuroscience from the Johns Hopkins University, completed his postdoctoral work at Rockefeller University and was faculty at the Burke Neurological Institute before moving to USMA.
Colonel Jason Barnhill currently serves as the Program Director of the Life Science Program in the Department of Chemistry and Life Science at USMA. As an infantry officer, he served as a mechanized infantry platoon leader and a light infantry company commander. As a lab officer in the Medical Service Corps, he has served as chief of clinical and research microbiology laboratories. He has a Ph.D. in Cell and Molecular Biology from the University of Hawaii.
© 2022 Hummel, Burpo, Hershfield, Kick, O’Donovan, Barnhill
The views expressed in this article are those of the authors and do not necessarily reflect those of the United States Military Academy, U.S. Army, Department of Defense, or U.S. Government.
Substantive Notes
[a] A biological toxin is defined as a chemical produced by metabolism in an organism, whereas a biological agent is a viral or bacterial pathogen and causes varying levels of health impacts based on lethality, transmissibility, and panic/social disruption. “Case Definitions for Chemical Poisoning,” Morbidity and Mortality Weekly Report, Centers for Disease Control and Prevention, January 14, 2005; “Bioterrorism Agents/Diseases,” Emergency Preparedness and Response, Centers for Disease Control and Prevention.
[2] Jennifer Doudna, “The promise and challenge of therapeutic genome editing,” Nature 578:7,794 (2020): pp. 229-236.
[5] David Cyranoski, “What CRISPR-baby prison sentences mean for research,” Nature, January 3, 2020.
[6] Martin Jinek, Krzysztof Chylinski, Ines Fonfara, Michael Hauer, Jennifer Doudna, and Emmanuelle Charpentier, “A programmable dual-RNA-guided DNA endonuclease in adaptive bacterial immunity,” Science 337:6,096 (2012): pp. 816-821.
[8] “Tularemia,” Johns Hopkins Bloomberg School of Public Health.
[11] Joint Publication 3-0, Joint Operations, U.S. Department of Defense, January 17, 2017.
[12] Army Doctrine Publication 3-0, Operations, U.S. Department of the Army, July 31, 2019.
[13] Ibid.
[14] Ibid.
[15] Daniel Sukman, “The Institutional Level of War,” Strategy Bridge, May 5, 2016.
[16] “Rajneeshee Bioterror Attack,” Homeland Security Digital Library.
[18] “Studying the Disease,” Centers for Disease Control and Prevention, updated July 1, 2020.
[20] Ibid.
[21] “State and Local Readiness: Medical Countermeasures,” Centers for Disease Control and Prevention, last reviewed October 5, 2020.
[22] Hummel, McNair, Burpo, and Bonner.
[23] “State and Local Readiness: Medical Countermeasures.”
[24] “FDA/DoD Collaborations,” Food and Drug Administration, current as of March 3, 2022.
[25] Hummel, McNair, Burpo, and Bonner.
[26] “Detect Novel Threats and Protect Force Readiness,” Defense Advanced Research Projects Agency.